Biophysical Analysis And The Development Of Follow-On Biologics
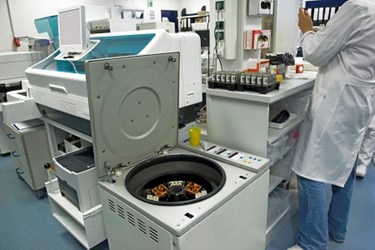
By Christopher Sucato and Mario DiPaola, Blue Stream Laboratories, Inc.
Introduction
The expiration of drug patents, and the subsequent approval of generic versions of the innovator product, is a long-established and well-understood pathway for developers of small molecule pharmaceuticals. Relatively more recent to the pharmaceutical industry is the end of the patent cycle for biopharmaceuticals, particularly recombinant protein drug products. The ends of these patents have given rise to follow-on biologics (biosimilars) as generic counterparts. Biologics hold a significant share of the pharmaceutical market. As late as 2013, biologics accounted for an estimated 22 percent of all pharmaceutical sales, and by 2023, the market share is expected to rise even further to 32 percent [1] . Furthermore, the current state in the biopharmaceutical industry is such that during the period between 2014 and 2018, some ten biologics with a collective estimated $60 billion in revenues will come off patent [2] .
However, due to the complexity of manufacturing biosimilars, as well as new regulatory processes, the biosimilar landscape is currently marred by uncertainty and in need of directive leadership. In this article we offer some historical perspective on biosimilars and the current state of the art. We discuss the challenges in sufficiently demonstrating biosimilarity, and in particular the importance that biophysical characterization of proteins plays in the development process.
The central focus of biophysical characterization is to gain information on the structural or conformational integrity of the protein entity comprising the drug product; that is, 1) the nature of the folded state of the polymer chain, 2.) how individual chains may associate to form higher order structures, and 3.) the ensemble of states that may be present in a specific sample type. By drawing attention to the important role of biophysical characterization, we hope to guide the interests of laboratories engaged in the development of biosimilars along a path towards an efficient and substantive platform for characterization of these complex products.
Biosimilars Outlook
The economic forces that drive the market for generic small molecule pharmaceuticals are amplified in the case of biologics, because of the high cost of development. The increasing demand, on a global scale, for recombinant protein biopharmaceuticals leads to rising costs and threatens to limit access to important therapies. For example, the annual cost of a typical Humira dosing regimen is estimated at over $20,000 [3]. Strikingly, the top six biologics in the U.S. consume almost half of the Medicare Part B drug budget [4]. Therefore, the introduction of biosimilars can, like small-molecule generics, have a positive effect on consumers and government healthcare agencies. This fact has been supported by recent legislation in the U.S. as part of the 2010 Patient Protection and Affordable Care Act. In conjunction with the new legislation, guidance documents by the FDA have been released to create a well-defined framework for biosimilars developers, regarding the analytical efforts towards demonstrating biosimilarity. The FDA guidance [5], along with the corresponding E.U. guidance [6], mandate the evaluation of physicochemical parameters, explicitly including protein higher-order structure.
Despite the guidance publications, however, global market and policy decisions are not yet fully clarified, and there may be questions and ambiguity regarding the development strategy around a biosimilar product. While a short list of biosimilars are currently approved for use primarily in Europe and, to a lesser extent, in the U.S., the development cost of biosimilars remains high compared to small molecule generics. Similarly, biosimilars currently do not offer the same level of cost savings to the consumer or subsidizing agency. As biosimilars become more commonplace, however, it is expected that policy decisions will be more resolved, resulting in a more systematic approach to biosimilar development and thus translating to higher savings in the cost of bringing this class of drugs to market. Biophysical characterization, we argue, is certain to grow in prominence as part of the systematic approach to biosimilar development that is taking shape.
The Challenges In Developing And Showing Biosimilarity
Recombinant protein-based pharmaceuticals offer great potential due to their highly targeted and specific intermolecular interactions and, thus, functionality which proteins afford. This advantage, however, comes at a cost in terms of development, because of the complex nature of a protein-based product compared to a small molecule drug. Biopharmaceuticals can be several hundred thousand kilodaltons in size, comprising many atoms and covalent bonds that are susceptible to variations in structure during the production process. They are also sensitive to environmental stress during purification, formulation, and storage. The effectiveness of protein-based pharmaceuticals depends on the proper linkage of amino acid residues and post translational modifications. A biologic’s efficacy is also dependent on a well-defined three dimensional structure that relies on the correct ordering of weaker, transient, non-covalent interactions between the protein and the solvent or formulation matrix, other proteins, and between parts of the same protein that may be far apart on the polymer chain. This exquisite non-covalent connectivity must be closely maintained during the production of the innovator product. The demonstration of a comparable higher-order structure for a biosimilar is a significant challenge.
Before any new pharmaceutical or generic is approved for marketing, its safety and efficacy must be proven in-vivo, ultimately in human clinical trials. However, before embarking into any in-vivo studies, it is essential to characterize the protein drug substance. To this end, primary sequence information and the extent of post-translational modifications are typically assayed using a combination of methods including chromatography, electrophoresis, and, especially, mass spectrometry techniques. To some extent, the primary sequence (including the post translational modifications) should contain all the information for folding, and fully determine the three dimensional higher-order structure of the protein drug substance. However, the final folded state of the protein can be sensitive to many parameters during expression, purification, and formulation. In fact, two protein drug substances with nearly identical primary structures can differ significantly in higher order structure because of their processing history. Biophysical techniques provide a direct measure of a protein's secondary, tertiary, and higher order structure — often directly in the formulation matrix. Therefore, such methods occupy an important middle ground between methods that allow for identification and characterization of the primary structure, and more late-stage studies for assessment of toxicity, immunogenicity, and efficacy.
How Can Biophysical Techniques Help In Demonstrating Biosimilarity?
The array of methods that fall under the category of "biophysical characterization", as it pertains to protein drug products, are primarily methods that interrogate the secondary, tertiary, or higher-order structure of the drug substance. While some laboratories may utilize high-resolution methods like multi-dimensional NMR, and even x-ray crystallography, the cost, complexity, and time investment of these methods can often preclude their widespread use. Rather, methods that drug developers often turn to are lower resolution methods that do not provide information at an atomic coordinate level. Instead, these provide structural information that is spatially averaged over the protein.
Low resolution spectroscopic methods depend on a reporter moiety of one form or another that relates to a structural characteristic of the protein, and ultimately has some correlation to the non-covalent bonding pattern of the folded polypeptide chains. Circular dichroism, fluorescence, and infrared spectroscopies fall into this category. Alternate approaches such as differential scanning calorimetry, analytical ultracentrifugation, and light scattering are also widely used to probe the non-covalent structural characteristics of protein samples. These approaches correlate the acquired experimental data with the non-covalent structural motifs within a protein, or the extent of non-covalent aggregates of several associated protein molecules.
The reporter moieties are not typically addressable to specific locations within the protein structure, and therefore the obtained information is spatially averaged. By using a combination of such methods, which rely on different reporters and different modes of measurement, it is possible to build an integrated picture of the protein structural motifs with significant advantages over any single high resolution technique. For example, larger proteins or assemblies can be assayed by low resolution methods often just as easily as smaller proteins or peptides, with no special effort or caveats. Many of the methods can be performed in a wide range of matrix conditions and can be used to study the protein stability as part of the product formulation development. Importantly, it is the combination of several different biophysical techniques that, as a whole, offer a complete picture of the protein’s conformational structure. We briefly discuss below some of the benefits and best practices pertaining to biophysical analysis of biosimilars.
Spectroscopic methods, as mentioned, are valuable for providing spatially-averaged structural information for the characterization of biosimilars in comparison to the innovator products. Circular dichroism (CD) spectra of proteins are particularly sensitive to the three dimensional orientation of the peptide bond group (far-UV CD; 190 to 250nm), disulphide bonds (near-UV CD; 250 to 320nm), or aromatic side chains (near-UV CD 250 to 290 nm). CD spectra in the far-UV and near-UV wavelength ranges can be used to quantify the types of secondary structure and the local folding environment around aromatic residues. Some drawbacks to CD, however, can be the limitations imposed by the type of buffers that can be used, due mainly to absorption in the far-UV wavelengths. Absorption by the protein itself also limits the concentration range that can be studied. In a comprehensive characterization, these limitations can be overcome by including an orthogonal method for secondary structure characterization. Fourier Transform infrared spectroscopy (FTIR), for example, should be included in a study of protein secondary structure. The absorption bands from stretching vibrations of the C=O (amide I) and C-N (Amide II) groups of the protein backbone can be used to quantify different types of secondary structure. Notably, FTIR is more sensitive than CD for beta-derived secondary structural conformations, which is useful in the characterization of beta-rich proteins like monoclonal antibodies.
While near-UV CD can be used to probe for similarity in the chiral environment around aromatic residues, fluorescence methods are also available to interrogate the local environment surrounding aromatic reporter groups. Using intrinsic tryptophan fluorescence, proteins may be characterized for the relative degree of solvent accessibility to tryptophan residues. Extrinsic fluorescence, for which the reporter is an exogenous dye that non-covalently interacts with the protein, can be used to measure the degree of solvent accessibility to hydrophobic pockets in general and in a wide variety of formulation conditions.
In addition to spectroscopic methods, other biophysical techniques should be employed to supplement biosimilar protein characterization and comparability to the originator product. Calorimetric techniques, in particular differential scanning calorimetry, provide information on the structural stability of the folded polypeptide. The temperature (Tm) at which denaturation occurs is a characteristic measure of protein stability directly related to the non-covalent interactions within the protein and between protein and the formulation matrix. Since the denaturation transitions should be the same for a protein drug product and its biosimilar, one can use the DSC thermograms to demonstrate that two products derived from different manufacturing processes are structurally comparable.
Protein aggregation is the process in which misfolded proteins accumulate into a non-native higher order associated state. There is evidence that such protein aggregation can result in enhanced immunogenicity [7] when administered in vivo. If the aggregation profile of a biosimilar can be shown to be different from the reference product, this may raise concerns about the possibility for immunogenic reactions with increased toxicity and possibly lower clinical efficacy [8]. Therefore, structural assays that have sufficient accuracy and precision for quantifying the higher-order associated states of protein drug products represent a crucial component in a biosimilar comparability platform of assays.
Determination of the soluble aggregate levels in protein pharmaceuticals has historically relied on size-exclusion chromatography (SEC). In recent years, however, there has been an understanding that SEC can provide inaccurate estimates of the aggregation profile [9] due to many factors, including 1) possible adsorption of the aggregates to the stationary phase; 2) analysis under non-native conditions, due to specific mobile phase requirements, thus affecting product profile; 3) physical filtration of large aggregates; 4) dilution effects resulting in disruption of weak aggregates [10,11]. While SEC-based methods continue to be relied upon, there has been an emphasis on identifying analytical techniques that can provide information on protein aggregate content using alternative separation approaches. Two frequently used orthogonal methods are analytical ultracentrifugation (AUC) and field-flow fractionation (FFF), both of which make use of instrumentation set-ups and, more importantly, separation mechanisms that differ from SEC. The value of an orthogonal method to SEC can readily become apparent from the protein size distribution determined by the different methods. In many cases, for example, AUC can provide key evidence that large soluble aggregates are present in a drug product, which are not observed by SEC for reasons as described above. AUC by contrast does not result in analyte dilution or capture on a stationary phase, and can often be done directly in the formulation matrix. Moreover, the precision and accuracy of AUC and FFF have evolved to the point where they can be used for accurately quantifying the aggregate content in support of biosimilar regulatory filings.
SEC and FFF methods are just two of several techniques available to the biophysicist for characterizing protein size distributions. No single assay, however, is able to quantify protein size distributions accurately over the full size range that may be present, and different methods can give conflicting results. Traditionally, the sub-visible particulate range is defined as that outside of the range measured by SEC (greater than roughly 0.1 μm), but smaller than what could be detected by imaging or counting methods, i.e. below about 100 μm. Within the sub-visible range, there may exist large protein aggregates that pose a risk of immunogenicity, and current guidance may not adequately address these risks for all drug products [12].
Dynamic light scattering (DLS) has been widely used to characterize micron and sub-micron sized particles in solution. The DLS measurement depends on the fluctuations in the intensity of light scattered by particles as they undergo Brownian movement. Based on this phenomenon, DLS can effectively quantify particle hydrodynamic diameters from 0.3 nm to a few microns. Therefore, because the dynamic ranges of many DLS instruments overlay well with the size of potentially adverse aggregate species, this method should be considered as a comparability test for any protein biosimilar characterization.
Conclusion
Biosimilars are quickly gaining position to be a cornerstone of the evolving pharmaceutical industry. Like other generics, these drug products can lower costs for life-saving therapies. But they must have an efficient and well-developed characterization platform in order to deliver on economic savings. Due to the complex nature of biologics, the three dimensional structure of the protein and the higher-order structural interactions must be taken into consideration. Biophysical techniques are geared for just this type of analysis, and therefore must be part of any biosimilar’s analytical characterization platform. The techniques we have outlined here, as part of an integrated package of biophysical assays, should form the foundation of the structural characterization of any biosimilar protein drug. Ultimately, these methods must be clearly delineated in revisions to guidance documents which, in turn, steer global pharmaceutical development policy.
[1] Pharmaceuticals: Going Large (2015, January 3rd) The Economist.
[2] Biosimilars Set to Boom as New Patent Cliff on Biologic Superstars Looms (2014, July 22nd) FierceBiotech.
[3] Japsen, B. (2011, May 24). Law change allows cheaper generic versions of biotech drugs. Chicago Tribune.
[4] MedPAC. Medicare payment systems and follow-on biologics, Report to the Congress: Improving Incentives in the Medicare Program. 2009.
[5] FDA Guidance Concerning Demonstration of Comparability of Human Biologic Products, including Therapeutic Biotechnology-derived Products (April, 1996) Center for Biologics Evaluation and Research (CBER), Center for Drug Evaluation and Research (CDER)
[6] Committee for Medicinal Products for Human Use (CHMP) Guideline on similar biological medicinal products containing biotechnology-derived proteins as active substance: quality issues (revision 1); EMA/CHMP/BWP/247713/2012; 22 May 2014
[7] M.P. Baker, H.M. Reynolds, B. Lumicisi, and C.J. Bryson, Immunogenicity of protein therapeutics: the key causes, consequences and challenges. Self Nonself. 2010 Oct-Dec; 1(4): 314–322.
[8] S. Deechongkit, K.H. Aoki, S.S. Park, and B.A. Kerwin., Biophysical comparability of the same protein from different manufacturers: a case study using Epoetin alfa from Epogen and Eprex. J Pharm Sci. 2006 Sep; 95(9):1931-43.
[9] J.F. Carpenter, T.W. Randolph, W. Jiskoot, et al., Potential inaccurate quantitation and sizing of protein aggregates by size exclusion chromatography: Essential need to use orthogonal methods to assure the quality of therapeutic protein products. J. Pharm. Sci. 99 2200-2208 (2010)
[10] T. Arakawa, D. Ejima, T. Li, et al., The critical role of mobile phase composition in size exclusion chromatography of protein pharmaceuticals. J. Pharm. Sci. 99 1674-1692 (2010).
[11] K. Tsumoto, D. Ejima, A.M. Senczuk, et al., Effects of salts on protein–surface interactions: applications for column chromatography. J. Pharm. Sci. 96 1677-1690 (2007).
[12] J.F. Carpenter, T.W. Randolph, W. Jiskoot, et. al., Overlooking Subvisible Particles in Therapeutic Protein Products: Gaps that may Compromise Product Quality. J Pharm Sci. 2009 Apr; 98(4): 1201–1205.
________________________________________________________________________________________________________________________________
1 Christopher Sucato is senior scientist and head of the biophysics group at Blue Stream Laboratories, Inc., Woburn MA 01801
2 Mario DiPaola is chief scientific officer at Blue Stream Laboratories, Inc., Woburn MA 01801.
2 To whom any correspondence should be addressed.