Cell Culture Trends To Watch In 2025
A conversation with Benjamin Bayer, Francisco Conesa BuendÃa, and Susan Sharfstein
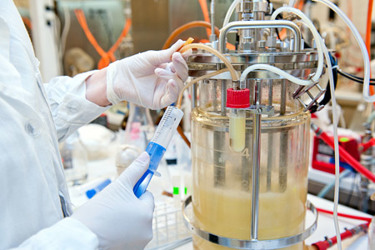
The advance of monitoring technology combined with more sophisticated cell line engineering methods is transforming the pharma industry’s approach to cellular biotechnology. This past year, we witnessed more uptake and improvement of tools like process analytical technology (PAT) and advanced cell line genome editing.
As 2024 comes to a close, we asked three upstream processing experts about what they’re looking forward to in 2025.
Process intensification: How do you see process intensification techniques, such as perfusion culture, continuous processing, or other novel approaches, affecting upstream manufacturing in the coming year? What are the potential benefits and challenges associated with these approaches?
I have high expectations that process intensification techniques, especially perfusion culture and continuous processing, will transform upstream biomanufacturing by enhancing efficiency (cost and timewise), reproducibility, scalability, and product quality. With careful planning, development and implementation, these methods could soon be more widely commercially viable.
However, realizing these benefits comes with challenges, particularly in process control, automation, and regulatory compliance. To address these, I see data science, PAT integration for real-time monitoring, automation, and bioprocess modeling (such as soft sensors and predictive models) as essential. Additionally, proper hardware, compatible software, and documentation are needed to support these advanced digitalized applications during operations.
Addressing these areas is crucial to unlocking the full potential of intensified biomanufacturing, paving the way for more flexible, reliable processes that meet rising market demands.
Benjamin Bayer, Ph.D., modeling expert
I expect that we will see increasing use of process intensification. At the Bioprocessing Summit, Amgen shared their experience with using both a new host cell line and new processes and I think they said they were getting 25 g/L.
The benefits included reduced costs, particularly capital costs, but ultimately process costs as well. In addition, smaller facilities allow for more flexibility in making a wider diversity of products. Challenges include the fact that perfusion and continuous processing are more challenging to implement.
Susan Sharfstein, Ph.D., University at Albany
Process intensification in upstream manufacturing, including perfusion culture and continuous processing, is set to enhance productivity, especially for high-demand biologics and cell therapies.
Perfusion culture maintains optimal cell growth by continuously removing waste and adding nutrients, allowing higher cell densities and productivity without larger bioreactors. Continuous processing integrates upstream and downstream operations, improving efficiency by reducing downtime between batches.
Benefits:
- Higher productivity: Supports high cell densities and faster cycle times, maximizing facility output.
- Enhanced quality: Continuous real-time monitoring and adjustments stabilize product quality.
- Cost and environmental savings: Cuts operational costs and raw material use, lowering environmental impact.
Challenges:
- Complexity and scalability: These techniques require advanced control systems, complicating scaling and implementation in multiproduct sites.
- Regulatory barriers: Current guidelines are batch-focused, so close collaboration with regulatory agencies is needed for compliance.
- High up-front costs: Specialized equipment and training require significant initial investment, which may limit adoption for smaller companies.
While these approaches promise cost-efficiency and consistency, overcoming technical, regulatory, and operational challenges will be essential for broader adoption in the next year.
Francisco Conesa Buendía, Ph.D., cell manufacturing expert
Single-use technologies: This year, manufacturers said reducing cost was their greatest wish for single-use products over any other new product development. What other circumstances do you see helping or hurting broader uptake of single-use systems?
Single-use systems have gained popularity in bioprocessing due to their flexibility, scalability for small batches, reduced cleaning needs, lower contamination risk, and ease of use in multiproduct facilities.
However, factors like supply chain resilience, environmental impact, and process scalability still affect their broader adoption, particularly for large-scale or eco-conscious production. Addressing these challenges, alongside advancements in PAT integration, standardization, and material innovation may alleviate these concerns, potentially making single-use systems more economically and environmentally viable in the long run.
Benjamin Bayer, Ph.D., modeling expert
Cell line engineering: What advancements in cell line engineering are you most excited about? How can we improve cell line productivity, stability, and robustness to enhance manufacturing efficiency and product quality?
I’m not an expert in advanced cell line engineering technologies like CRISPR-based gene editing, vector design, synthetic biology, or adaptive laboratory evolution. However, I believe significant progress in enhancing manufacturing efficiency already can be made by optimizing existing approaches for selecting high-producing clones. This includes analyzing various markers during clone screening, such as carbohydrate and amino acid consumption patterns.
Using multivariate analysis to select high-producing clones can lead to higher yields, improved consistency/stability, and robustness across scales by providing a more complete view of clone physiology. Often, this straightforward yet more labor-intensive step is overlooked in favor of simply identifying the highest producers in a certain process scale. Combined with high-throughput systems, like miniaturized parallel bioreactors, many clones can be screened simultaneously, and even online data can be considered. This approach helps select high-producing clones exhibiting proper cell physiology, while identifying and excluding initially promising clones with problematic metabolic profiles or high oxygen demands, avoiding later issues in large-scale production.
In summary, I believe fully leveraging existing knowledge of cell physiology is essential before adopting new cell line engineering technologies. Additionally, such a solid multivariate evaluation subsequently further supports more advanced methods in cell line engineering.
Benjamin Bayer, Ph.D., modeling expert
One exciting advancement in cell line engineering is the use of multiplexed CRISPR editing, which enables simultaneous modifications across multiple genes to optimize cellular performance. This approach allows us to enhance productivity by targeting pathways that improve nutrient uptake and reduce metabolic waste, directly boosting yield and stability.
Additionally, advancements in adaptive stress conditioning help develop cell lines that are pre-adapted to withstand manufacturing stresses, reducing variability and improving robustness. Synthetic biology tools are also allowing us to design “smart” cells capable of sensing and responding to changes in their environment, which helps maintain consistent growth and productivity. Together, these innovations are making cell lines more efficient, stable, and suited for high-quality biomanufacturing.
Francisco Conesa Buendía, Ph.D., cell manufacturing expert
I am most excited about targeted integration. At a meeting I attended recently, Pfizer said that using their targeted integration method, they screen about a dozen clones. On our research side, we are working on ‘omics analyses of high- and low-productivity cell lines and collaborating with (yet another) small business looking at using a novel codon optimization approach to improve productivity with a possibility of cell line engineering either to alter tRNA levels or to engineer in codon-optimized transcription factors.
Susan Sharfstein, Ph.D., University at Albany
Real-time monitoring and control: How can we use real-time monitoring and control technologies to optimize upstream processes and improve product consistency? Looking at the year ahead, what specific parameters are you focusing on for real-time monitoring and control?
Real-time monitoring and control are essential for optimizing upstream processes and improving product consistency, particularly in cell manufacturing and advanced therapy medicinal products, where process variability impacts product quality.
Key approaches:
- PAT: Integrates sensors for real-time data on pH, dissolved oxygen (DO), glucose, and cell density, enabling immediate adjustments to maintain optimal conditions.
- Automated control systems: Automatically adjust inputs like nutrient feed and oxygen in response to real-time data, stabilizing the cell culture environment.
- Predictive analytics: Machine learning on real-time data anticipates process variations, allowing proactive control.
Parameters of interest to take into account:
- Metabolite levels: Real-time glucose and lactate monitoring helps balance cell health and prevent toxic buildup.
- Cell viability and density: Ensures healthy cell growth, critical for product consistency.
- Dissolved oxygen and pH: Automated control maintains optimal conditions, reducing stress-induced variations.
- Product quality attributes: Inline technologies like Raman spectroscopy monitor quality markers in real time.
- Temperature and agitation: Maintains uniform conditions, reducing cell damage and variation.
By focusing on these parameters, real-time monitoring and control enable tighter process consistency, enhancing product quality and yield.
Francisco Conesa Buendía, Ph.D., cell manufacturing expert
Integrating advanced PAT for real-time monitoring and control has immense potential to transform upstream bioprocessing. These technologies allow real-time tracking of key process variables, which are hard or impossible to measure directly inside the bioreactor, enabling precise control of critical parameters in a timely manner. This capability, monitoring important process variables as well as performing process steps at critical phases instead of predetermined time points, which was previously unavailable, leads to higher yields, improved product consistency, and reduced batch variability, resulting in fewer rejected batches.
A primary focus will be on real-time monitoring of viable cell density, biomass, and nutrient and metabolite levels, such as glucose, lactate, and ammonia. Probes like biocapacitance, Raman, and near-infrared spectroscopy enable continuous tracking, allowing for continuous tracking of these parameters and dynamic control of cell growth phases with automated, cell-specific process and feed adjustments. These approaches support maintaining optimal cell growth, minimizing toxic byproducts, and prevent nutrient imbalances, which is especially beneficial in intensified processes like perfusion or high-density fed-batch, where maintaining high cell viability and consistent biomass is crucial for high productivity.
Over the coming year, emphasis will be on leveraging and integrating these advanced sensors, along with soft sensing and predictive data analytics, to maximize their potential. Together, these technologies are enabling highly controlled, automated, and efficient bioprocesses that minimize variability and enhance product quality.
Benjamin Bayer, Ph.D., modeling expert
We are not really doing this in my lab, but my wish list includes real time analysis of product quality attributes. I would like to give a shout out to the folks at 908 Devices who have made amino acid analysis much faster and have added the possibility of at-line measurement.
Susan Sharfstein, Ph.D., University at Albany
Supply chain resilience: In light of recent supply chain disruptions, what strategies are you implementing to ensure the resilience of upstream manufacturing operations? Beyond redundancy, how can we mitigate potential risks and maintain the uninterrupted supply of critical raw materials and reagents?
Technologies like PAT integration, soft sensing, and bioprocess modeling offer essential tools for building resilience in upstream manufacturing amid supply chain disruptions. They help conserve raw materials, while enabling advanced biomanufacturing with greater adaptability, optimization, and risk management. For example, PAT and soft sensing enable smarter process control and automation, reducing batch variability and manual intervention.
Additionally, (predictive) models and digital twins allow for in-silico experimentation, which reduces the need for wet-lab experiments, saving materials, cutting costs, and accelerating timelines. Together, implementing these analytical and modeling approaches will strengthen manufacturing resilience, reducing material use, avoiding batch failures, and supporting steady production despite supply chain challenges.
Benjamin Bayer, Ph.D., modeling expert
This is not something we do. I am, however, pleased to see that we can now get nitrile gloves, disposable plastics, etc., again without any delays.
Susan Sharfstein, Ph.D., University at Albany
About The Experts:
Benjamin Bayer has more than eight years of scientific experience working in upstream bioprocess modeling, PAT tools, and data science in academia, the startup sector, and industry. His work focuses on developing and integrating advanced modeling tools to facilitate bioprocess development, characterization, optimization, and advanced process control strategies.
Francisco Conesa Buendía, Ph.D., has been working in the field of advanced therapies since 2021. His research focuses on the development of ATMPs based on stem cells and chondrocytes. Currently, he is a cell manufacturing assistant working on manufacturing and process optimization of cell and gene therapies based on CAR-T and T cells at Memorial Sloan Kettering Cancer Center.
Susan Sharfstein, Ph.D., is a professor at the University at Albany’s College of Nanotechnology, Science, and Engineering. She leads the Sharfstein laboratory, which is focused on the role of culture conditions and cell physiology and the use of living systems for industrially relevant processes.