Comparison Of WFI Production Methods: Multi-Effect Distillation Vs. Vapor Compression
By Sandeep Desai, independent subject matter expert
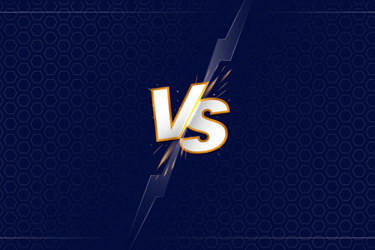
There are a number of pharmaceutical applications where pure, distilled water is necessary to avoid the risk of contamination and to ensure that no additional compounds react with the water for potentially adverse impacts. Parenteral pharmaceuticals are a major example of such products. These are administered directly into the circulatory system or tissue via injection. Their usage began back in the 19th century, particularly in 1830, when Moscow reported the initial intravenous attempts. Early failures dictated that there was a need for further research, which found that the primary issue was the water base being used for administration. It highlighted the need for ultrapure water for injection (WFI).
The development of distilled water for pharmaceutical use then became a primary focus for researchers. The goal was to develop a method that would ensure consistent results and high yields, all while reducing the need for constant investment in equipment. Governing bodies also found that there were several instances where different water distillation processes yielded different purity levels, leading to the formulae reacting differently in patients. Therefore, WFI standards were developed, which got stricter with the passage of time and evolution of medicine. The leading authority in this regard is the United States Pharmacopeia (USP). It governs several critical parameters, such as:
- total organic carbon (TOC),
- conductivity, and
- endotoxin levels.
These factors all help mitigate the risk of introducing microbial or chemical contaminants into pharmaceutical products and, in turn, the human body. For example, WFI produced in bulk must ideally contain less than 500 micrograms of organic carbon per liter. The bacterial endotoxin limit in WFI is set below 0.25 EU/mL.1
There are multiple processes for producing WFI in bulk (hot and cold). The two most common hot WFI generation methods are multi-effect distillation (MED) and vapor compression (VC).2 While both processes can produce WFI as per USP and other pharmacopeia requirements, there are significant differences in terms of operational principles, energy efficiency, and system complexity.
Multi-Effect Distillation (MED) Process
Figure 1: MED Process
Figure 1 shows how MED employs latent heat generated from steam in effect 1 to drive evaporation in subsequent chambers. Since the steam from one effect transfers to the second one, thermal energy conductivity dictates better water purity via repetitive evaporation-condensation cycles. The energy is conserved from one effect to another, pushing water through multiple evaporation/condensation cycles across each effect. The more effects there are, the higher the purity. However, more effects lead to a trade-off between purity and energy efficiency. As the number of effects increases, so does the energy needed. Generally, three effects are enough to achieve standard sufficient purity. However, competitive differences and other specific requirements may also require additional chambers.
The MED process begins with two key inputs, feedwater and steam. Steam is introduced into the heat exchanger first to prime the unit for distillation. Then, feedwater — preferably pretreated — is introduced into the first effect. This pretreatment is done to ensure initial purity and is a standard requirement. The feedwater is sprayed as a fine spray over heat exchanger tubes through which the steam flows.3
The steam transfers its thermal energy directly to the feedwater. Because of the fine spray, the particles are usually sized between 100 and 400 microns, which means they get heated much quicker, causing the feedwater to evaporate into vapor. The vapor leaves behind all impurities, evaporating in a pure form. Furthermore, the heated vapor becomes the heat source for the next effect.4 The condensation that results is collected as WFI output from the first chamber, into the next one. The process takes place all over again, further improving the water purity. The distilled water becomes the output of the first chamber and the input (feedwater) of the second chamber.3
What makes MED “multi-effect” is that the vapor from chamber/effect-1 is fed into chamber/effect-2 as its heat source (steam). And the condensed vapor (water) is introduced as the feed water into the second effect. The process continues for the number of effects there are in the system.3,5
It is important to note that the waste, i.e., liquid that contains all the contaminants, isn’t evaporated. It must be removed from each effect after each cycle. The MED process relies heavily on the hierarchical arrangement of effects, as shown in Figure 1. As they subsequently come into play, the temperature and pressure therein should also be lower. This is to ensure energy conservation, as the thermal energy in the vapor from one effect is sufficient to evaporate the feedwater in the next effect without the need for additional heat input. A larger drop is needed for every chamber to drive the phase change and ensure high thermal efficiency.
The difference can be calculated with a simple Darcy-Weisbach equation.6
Where:
- ΔP is the pressure drop (Pa)
- f is the Darcy friction factor (dimensionless)
- L is the pipe length (m)
- D is the pipe diameter (m)
- ρ is the fluid density (kg/m³)
- v is the fluid velocity (m/s)
The equation may need further changes based on different MED designs.
The output purity comes from the repetition of the evaporation/condensation cycles. The feed water goes through several evaporation/condensation cycles and, at each stage, it leaves fewer and fewer impurities behind.
Below is the process map for a commercially available MED for WFI.
Figure 2: Processing Map
Routine Operation and Maintenance
MED systems are relatively complex compared to their VC counterpart. One of the most complicated aspects therein includes the cleaning protocols designed to help prevent the buildup of scale and biological contaminants in the heat exchangers and piping systems.
There are several maintenance tasks that are necessary for MED, including:
- regular descaling,
- inspection of heat transfer surfaces,
- cleaning of heat exchanges,
- spray nozzle clear up,
- distilled water collection cleanup and sanitation,
- pressure and temperature regulator calibration, and
- calibration of other control systems for efficient flow of heat, vapor, and distilled water collection.
The complexity of routine operation and maintenance of MED plants is usually because of the interdependencies in the system. A failure in any one aspect in any of the effects could lead to the failure of the entire system. This risk is mostly due to the parallel nature of MED effects.7
Operational and Capital Costs
While energy efficient, MED systems are usually more cost-effective than VCs. The price for small MED systems may range from $8,000 to $12,000, while larger industrial plants can cost much more. Furthermore, compared to VC, MED systems usually have a larger footprint.5 Each subsequent chamber further increases its footprint and cost due to the associated heat exchangers, pumps, and controls.
For long-term operational costs, primarily driven by steam consumption, the energy reuse of the MED system makes it much more viable for large-scale WFI production compared to others. This is particularly the case due to the relatively lower operational costs for water distillation and desalination. Reverse osmosis (RO) plants usually cost 20 cents/m3 to operate, while MED plants cost 39 cents/m3.8 However, the water quality and purity of an RO plant does not always meet the WFI requirements for pharmaceutical use in many cases and requires repeated passes, which defeats the cost-effectiveness of the process.
Vapor Compression (VC) Process
Figure 3: VC Process
Another popular WFI generation system is the VC method. If differs from MED in a number of ways, with the most prevalent one being that instead of using the steam in heat exchangers, it utilizes mechanical energy to generate pressure and temperature in the system. This, in turn, reduces the amount of external heat required for the process. As Figure 2 shows, it has a built-in compressor that increases the pressure of the steam, heating up the coils needed for feedwater evaporation. This energy- and preparation-efficient design is what makes VC systems a popular choice for WFI production for many labs.
The VC process starts with preheated water or steam being introduced into the system to prime it, similar to MED. This primes the machine, starting the compressor up. The feedwater is then sent in again as a spray. As the compressor starts up, it pressurizes the preheated water or steam, further increasing its pressure. This means that the priming input for VC doesn’t need to be as hot or energy intensive as for MED.
The feedwater goes through multiple preheaters and heaters (typically electrical) that evaporate the water. This is fed into a compressor that energizes the vapor, increasing both the pressure and temperature. That steam is fed to chambers or pipes while more feedwater is introduced into the system. The steam heats up this new water, turning it into steam to be fed into the compressor while it cools down and is condensed into the output WFI.
This heat transfer between the compressed vapor and incoming feedwater is the primary functionality behind the VC process. The evaporating feedwater condenses on the top, leading to distilled water being collected as WFI underneath. Since the process is significantly cyclical, it allows for better energy recycling, reducing the need for constant heat input as in different effects of the MED. Furthermore, it also makes VC systems much more energy efficient than single-stage distillation methods.
Similarly, the condensation part of the process has an enormous impact on the purity of the WFI collected. The use of high-grade metals to prevent metal ion contamination despite high temperatures, employing highly polished construction (to avoid microscopic crevices that can lead to bacterial growth), minimizing/eliminating dead spaces that can be breeding grounds for microbial growth, and ease of sterilization are just some of the aspects of good construction that can lead to sufficiently high output purity.
The core functionality in the VC process revolves around its compressor. The compressed vapor increases the vapor’s enthalpy, making it an efficient heat source for the incoming feedwater. Further operations are actively driven by the thermal recycling of the VC system, hence setting it apart from other processes like MED.
A key factor herein is the type of compressor used. The most common types of compressors used for this include:
- centrifugal compressors,
- axial flow compressors, and
- rotary screw compressors.
Materials used in the construction of the compressor and associated piping — such as high-grade stainless steel — are also crucial for preventing contamination and ensuring the purity of the final WFI product.
Routine Operation and Maintenance
Due to the lower number of operational parts and effectively working with only one chamber, VC systems generally have lower operational and maintenance complexity. Compared to MED systems, they are easier to clean and maintain. However, the compressors in VC systems require regular inspections because the heat leads to general wear and tear of compressors, especially after extensive usage. The bearings may expand with the heat, leading to damage to the moving components of the compressor. Furthermore, the seals may rupture, and seals may be subject to rust due to the humid operating environment, hence requiring frequent replacement to maintain efficiency.
As with any other distillation plant, the heat exchanger and base plate must also be cleaned for prevention against scaling and fouling due to water contaminants. If allowed to settle, the base plate may need replacement. Otherwise, this could lead to reduced heat transfer and, therefore, poor efficiency. There is also the risk of reduced purity of the distilled water as a result.
It is important to note that VC systems usually operate under much higher mechanical loads than MED systems. The pressure valves, pipes, and heat exchangers must therefore be regularly maintained to avoid the risk of failure or disaster due to the high pressure.
Operating Costs
VC systems are usually more efficient in terms of energy consumption due to their ability to recycle heat more efficiently compared to MED. However, they tend to be priced higher than MED as well. For smaller pharmaceutical operations’ WFI needs, a standard system may cost $10,000 to $25,000. The major consideration for this price range includes the materials it is made of and, more importantly, the compressor’s build.
However, while the initial cost is high, VC systems usually offer better operational costs due to better energy conservation. The system recycles the latent heat of vaporization with the help of mechanical compression. Furthermore, the need for external steam or electrical heat inputs is considerably lower. Each cycle can cost as low as 15 cents/m3.
However, it is important to note that due to the multiple-effect operation of MED, the yield after only two effects is much purer in MED compared to VC. This means that VC needs to operate longer for similar yields, with operational costs going as high as 50 cents/m3 to $2/m3 with high-performance compressors and heat exchangers.
Detailed Comparison Of MED Vs. VC For WFI Production
The two processes differ in much more than just mechanisms and technology used. Understanding these differences can help pharmaceutical businesses make informed decisions about which equipment (and process) to use for WFI production.
Table 1: MED vs. VC comparison overview
Output Quality Parameters For MED And VC
The output quality of WFI is governed by several pharmacopeial standards, primarily those established by the USP, European Pharmacopeia (EP), and Japanese Pharmacopeia (JP). The quality of WFI generated by both MED and VC processes is subject to stringent guidelines, particularly with regard to purity, conductivity, microbial content, TOC, and endotoxin levels. Both MED and VC are widely adopted for WFI generation because they consistently meet these criteria, although there are nuanced differences in the output quality that each process can deliver, depending on factors such as system design, operational parameters, and the nature of feedwater pretreatment.
Table 2: MED vs. VC Output Quality Parameters
Regulatory Preferences
The USP approves of two methods of producing WFI — distillation and RO — and the FDA endorsed this in a 2016 memo. It also acknowledges the use of ultrafiltration (UF) but only for a specific part of WFI production, i.e., endotoxin reduction. Since 2017, the European Pharmacopeia also has allowed RO for WFI production. Previously, it was just distillation, something both MED and VC adhere to.
This shows that regulatory authorities consider both MED and VC preferred methods of WFI production. Up until a few years ago, the lean was toward MED, but now the core focus is achieving desired water quality and adhering to parameters like TOC, conductivity, bacteria, and endotoxin limits.
Conclusion
Considering VC's significant edge over MED in almost all comparison dimensions, especially if it can achieve comparable or even better water quality parameters by introducing other elements to the WFI generation systems, VCs may be the right choice for most pharmaceutical producers. Traditionally, MEDs had a much higher output capacity, but now most VC systems may have a similar or higher maximum output capacity compared to MED systems from the same vendors.
References
- P. Mazzola, "Chemical resistance of the gram-negative bacteria to different sanitizers in a water purification system," BMC Infectious Diseases, vol. 6, no. 1, 2006.
- J. C. Ankers, "Water for the CIP System," Drugs and the Pharmaceutical Sciences, vol. 173, 2008.
- G. C. M. O. A. Idris, "Incorporating potential environmental impact from water for injection in environmental assessment of monoclonal antibody production," Chemical Engineering Research and Design, vol. 109, pp. 430-442, 2016.
- M. A. A. J. H. L. Karan H. Mistry, "An improved model for multiple effect distillation," Desalination and Water Treatment, vol. 51, 2013.
- J. Li, X. Wu, J. Zhang, and L. Wang, "Economic evaluation of 20,000 M³/day seawater desalination coupling with floating reactor nuclear power plant," IOP Conference Series: Earth and Environmental Science, vol. 352, 2019, pp. 1-10.
- J. Mousdell, "Hazen-Williams vs. Darcy-Weisbach," 2021. [Online].
- H. Kuhlman and D. Coleman, "Engineering aspects of WFI systems design," in Sterile Pharmaceutical Products, 1st ed., D. K. Siahaan, Ed. New York, NY: Routledge, 2018, pp. 221-268.
- J. Manfredi, "Water systems for parenteral facilities," in Parenteral Medications, 4th ed., W. G. Elmer, Ed. Boca Raton, FL: CRC Press, 2019, pp. 653-672.
- S. Fishkin, "High purity water," in Handbook of Downstream Processing, Springer, Dordrecht, 1997.
- S. K. Ashish Baldi, "Water for Pharmaceutical Uses: Quality Control and Validation," in Water for Health, 2013.
- .C. L. W. K. L. M. Lixiong Li lli, "Kinetics of Hydrothermal Inactivation of Endotoxins," Applied and Environmental Microbiology, vol. 77, no. 8, 2011.
- F. Rögener, "Increasing the Sustainability of Pharmaceutical Grade Water Production," Chemie Ingenieur Technik, vol. 96, no. 4, 2024.
- D. M. R. R. A. N. B. B. G. S. S. S. S. R. S. Gousia Begum, "A Review On Formulation And Evaluation Of Parenterals," Indian Research Journal of Pharmacy and Science, 2018.
- Aqua-Chem, Vapor Compression Distillation Vs. Multiple Effect Distillation.
- M. I. M. B. Yigit Kupcu, "Exergy analysis of purified water plant in a pharmaceutical Industry," International Journal of Exergy, vol. 40, no. 1, pp. 34-43, 2023.
- Meco, A Look at the Cost of Alternative Systems for Producing Water for Injection (WFI) Including Membrane-Based WFI Production Absent Distillation, 2021.
About The Author:
Sandeep Desai has 20+ years of experience in pharmaceutical engineering, specializing in HVAC systems, water systems, and manufacturing process equipment. With years of experience in facility design, qualification, and process optimization, he has implemented advanced HVAC solutions to maintain classified environments and ensure regulatory compliance. Adjacent experience lies in designing, installing, and optimizing purified water, WFI, and clean steam systems to meet stringent pharmaceutical standards. He has led modifications and enhancements in filling lines, lyophilizers, autoclaves, and sterilization systems to improve efficiency, reliability, and product integrity. In previous roles, he has worked with OSD and ointment facilities.