Do We Need Comparative Clinical Efficacy Testing For Biosimilars?
By Sarfaraz K. Niazi, Ph.D., adjunct professor of pharmaceutical sciences, University of Illinois at Chicago
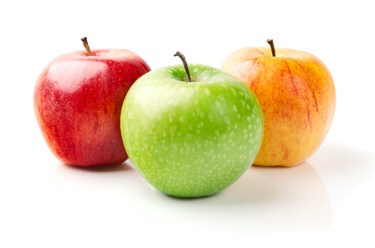
Of the 100+ biosimilar products approved by the FDA and EMA, all, except a couple, provided evidence of comparative clinical efficacy. Surprisingly, while the pharmacokinetic (PK) studies of biosimilars failed for several products, none of the comparative clinical efficacy tests failed. In all those instances where the pharmacokinetic studies failed, the developers were allowed to repeat the studies using a different population until they met the equivalence criteria. No biosimilar application was rejected due to failing pharmacokinetics studies in the first attempt or for failure to show clinical equivalence in comparative testing. The FDA, in its Biosimilar Action Plan (BAP) and its recent analytical assessment guideline, as well as the many statements by FDA leaders, emphasizes a need to assess whether comparative clinical efficacy testing provides confidence in the safety and efficacy of biosimilar products. Alternate methodologies like in situ pharmacokinetic modeling have also been proposed by the FDA. This article examines the nature of comparative efficacy testing and suggests that these studies may be providing misleading evidence of safety and efficacy of biosimilars and should be replaced with more objective pharmacokinetic studies.
Background
With almost 400 currently approved biological therapeutic products,1 it is anticipated that most of these products will be introduced as biosimilars once the biologics’ patents expire. Given the diversity of these products, it has been difficult for the regulatory agencies to provide general guidance for development and, historically, both developers and agencies continue to rely on comparative safety and efficacy studies for the approval of biosimilars. However, an analysis of the FDA-licensed biosimilars2 shows that several of the 50 or more reported clinical pharmacology tests failed, yet none of the over 40 clinical testing reported in patients failed.3 Three products — Retacrit (erythropoietin), Nivestim (filgrastim), and Udenyca (pegfilgrastim) — were licensed without testing in patients. The regulatory agencies are seeking alternatives to clinical efficacy testing after establishing that the finding of comparable efficacy cannot overrule unexplained differences in a pivotal PK study.4
In July 2018, the FDA issued a Biosimilars Action Plan5 that proposed using an in silico modelling and simulation approach to correlate pharmacokinetic and pharmacodynamic responses with clinical performance, with the goal of obviating clinical efficacy testing. An examination of the European public assessment report (EPAR)6 guidance on 84 authorized biosimilars, in which two applications were rejected and 34 withdrawn, convinced the EMA that some biosimilars do not need clinical efficacy testing (e.g., teriparatide, insulins, low-molecular-weight heparins, filgrastim, and pegfilgrastim and other cytokines with PD markers). Some products would not even need safety assessments, such as teriparatide, low-molecular-weight heparin, and insulins. The FDA has licensed erythropoietin, pegfilgrastim, and filgrastim products without requiring comparative efficacy testing.
This article proposes changes to testing protocols of clinical pharmacology testing to obviate the need for clinical efficacy testing, in line with the new thinking of the FDA and EMA.
Limitations Of Comparative Clinical Efficacy Testing
According to the FDA, “As a scientific matter, a comparative clinical study will be necessary to support a demonstration of biosimilarity if there are residual uncertainties about whether there are clinically meaningful differences between the proposed and reference products based on structural and functional characterization, animal testing, human PK and PD data and clinical immunogenicity assessment.” The FDA has licensed 26 biosimilar products7 for which multiple clinical efficacy testing was conducted. Does it mean that there was sufficient “residual uncertainty” to require testing in patients? A more pertinent question is, does a single comparative efficacy test remove all residual uncertainty across many indications?
Since biosimilar trials are conducted as equivalence (or non‐inferiority) trials,8 the equivalence margins are crucial for the testing decision and need to be prespecified. In efficacy trials for a novel drug, the studied indications are diverse. As the margins have to reflect a difference that is not relevant from a clinical point of view, the acceptable difference depends on the disease. This makes it impossible to use a standardized margin, like the bioequivalence testing margin.9 The equivalence margins in efficacy trials must be clinically and statistically justified.10 A review of the EPARs and BLAs of approved biosimilar products shows extreme diversity in the selection of margins and whether they were prespecified, such as in the case of Omnitrope,11 Blitzima,12 Ritemvia,13 Rituzena,14 and Truxima.15 In some cases, the equivalence margin was chosen post hoc and justified by using historical data.
Adalimumab products Amgevita16 and Solymbic17 used a margin of 0.738 and 1.355 for ACR20 responders risk ratio, while Imraldi18 used a margin of 15 for ACR20 responders and Cyltezo19 used a margin of -12 and 15 for ACR20 responders at 12 weeks. However, the adalimumab “similar biologic” approved in India (Exemptia) used an equivalence margin of 28.5 percent, allowing for a much smaller sample size and a margin of 23 percent for an infliximab similar biologic.
The noninferiority trials do not exclude the possibility of increased activity of the biosimilar product that may be associated with more adverse events. Several noninferiority studies have failed, but the agencies ultimately approved these molecules. Such was the case of trastuzumab biosimilars, where the upper bound of the predefined equivalence margins for the primary endpoint (pCR), while confirming noninferiority, did not exclude the possibility of superior efficacy.
The subjective nature of comparative efficacy testing leaves much to doubt about the utility of these studies in overcoming any deficiencies in the stepwise testing, from analytical assessment to clinical pharmacology testing.
Utility Of Clinical Pharmacology Testing
The clinical pharmacology testing of biosimilars is based on the bioequivalence model of generic chemical products, in accordance with CFR 21 320.22.20 However, according to this regulation, a drug product's in vivo bioavailability or bioequivalence may be considered self-evident if it is a parenteral solution for administration by injection, or an ophthalmic or otic solution, and contains the same active and inactive ingredients.
In the case of biosimilars, the testing is required regardless of these waivers allowed to chemical generics, for a variety of reasons. First, the bioavailability of biosimilars varies despite their administration via parenteral routes. This was demonstrated for subcutaneous administration of filgrastim, where a three-fold increase of AUC0-t and Cmax was observed when the dose was increased from 5 to 10 μg/kg — a two-fold increase. When administered intravenously, the parameters were proportional to the dose. The absolute bioavailability of the subcutaneous dosing of XM02, a filgrastim product, was 33 and 45 percent at the two doses, respectively.21 For most biological products, there is a significant dose-response correlation, making the bioequivalence studies essential to conduct.
Another reason why the pharmacokinetic studies are useful comes from the comparison of the disposition profile, which describes the distribution and elimination of drug. The disposition profile can be altered by subtle structural differences, making the pharmacokinetic studies a powerful tool to support the analytical assessment of the biosimilar product.
The utility of clinical pharmacology testing can be improved by taking a scientific approach to these studies as proposed below:
- The bioequivalence testing model22 concludes bioequivalence between the two treatments if the 90 percent confidence interval of the ratio of a log-transformed exposure measure (AUC and Cmax) falls entirely within the acceptance range of 80 to 125 percent. However, given the diversity of molecular structure and structural variance, it is reasonable to suggest that the agencies should not require a one-size-fits-all approach.23
- If the prespecified and justified equivalence boundaries are not met, the applicant is required to explore root causes and adequately reflect the results in the planning and conduct of a subsequent PK study. In addition, sound justification must be presented as to why a new PK study showing equivalence can overrule the previous failed study. There were several failed PK studies reported in the EPARs: Cyltezo (adalimumab), Hyrimoz (adalimumab), Ziextenzo (pegfilgrastim), Terrosa (teriparatide), Grastofil (filgrastim), and Efgratin (pegfilgrastim). All of these products were retested with different study populations and study power until they passed the comparison. One way to obviate protocol-dependent failures is to select the study population differently than it is done for the new drug development. The acceptance criterion of the study population can be narrowed to reduce variability, thus reducing the number of subjects required; as an example, this may include a population of males 18 to 36 years old, nonsmokers, with BMI of 20 to 23.
- The bioequivalence testing model is more sensitive to bioavailability, whereas in the case of biosimilars, the disposition profiling is more critical; the statistical testing of pharmacokinetic data can be more robust by including additional parameters like the distribution volume (Vd) and elimination rate constant (Kel) that are more sensitive to subtle differences in the structure of the molecule. The concept of distribution volume as a function of time was developed by the author,24 and it has been widely used in developing in silico pharmacokinetic models to correlate efficacy with the pharmacokinetic profiles.25 Differences in the distribution kinetics due to binding variation affect the total Vd, as well as the change of Vd as a function of time, providing a valuable comparison opportunity between a biosimilar candidate and the reference product. The distribution volume of a drug is highly sensitive due to following factors:
- Molecule size: The larger the molecule, the slower the passive diffusion is and, therefore, the smaller the Vd.
- Molecule charge: Highly ionized charged molecules will have higher water solubility and will be held in the central compartment by electrostatic factors, resulting in higher binding to central compartment proteins.
- Tissue binding: Tissue binding reduces chemical activity, forcing more molecules to leave the blood compartment into deeper tissues.
- pKa: pKa determines degree of ionization and, therefore, the lipid solubility.
- Lipid solubility: Highly lipid-soluble drugs have higher Vd because of low fat in the blood.
- Water solubility: Difficult lipid bilayer membrane transport and smaller Vd and remain in the extracellular fluid.
- Since the disposition profiles are more sensitive to structural differences, binding to tissue, and metabolism, dose-dependent testing is required to assure that the differences in the disposition profiles are not missed because of the nonlinearity of disposition parameters. Generally, two doses — one clinical and one half the clinical dose — will be needed to make the evaluation more robust.
The in silico approach to pharmacokinetic modeling may allow regulatory agencies to have greater confidence in the comparability of total body exposure and, therefore, the efficacy of biosimilars, as the FDA has represented in its Biosimilars Action Plan.
Conclusion
Clinical testing of biosimilars in patients does not necessarily establish proof of safety and efficacy and, in most instances, does not constitute a resolution of "residual uncertainties" in a biosimilar product. A modified in silico approach to clinical pharmacology testing offers a better choice to establish definitive proof of biosimilarity. More studies and simulations are needed to establish the full utility of clinical pharmacology data, to avoid the risk of approving biosimilars based on limited efficacy testing that may not have appropriate biosimilarity.
References:
- http://crdd.osdd.net/raghava/thpdb/
- https://www.fda.gov/drugs/biosimilars/biosimilar-product-information
- https://www.accessdata.fda.gov/scripts/cder/daf/
- https://www.sciencedirect.com/science/article/pii/S1865921718302009#bib0130
- https://www.fda.gov/media/114574/download
- https://www.ema.europa.eu/en/search/search/field_ema_web_categories%253Aname_field/Human/ema_group_types/ema_medicine?search_api_views_fulltext=biosimilars
- https://www.fda.gov/drugs/biosimilars/biosimilar-product-information
- https://www.ncbi.nlm.nih.gov/pmc/articles/PMC3019319/
- García‐Arieta A, Gordon J. Bioequivalence requirements in the European Union: critical discussion. AAPS J 2012; 14: 738—748. [PMC free article] [PubMed] [Google Scholar]
- CHMP. Guideline on similar biological medicinal products containing biotechnology‐derived proteins as active substance: non‐clinical and clinical issues (revision 1). 2015. Available at http://www.ema.europa.eu/docs/en_GB/document_library/Scientific_guideline/2015/01/WC500180219.pdf
- https://www.guidetopharmacology.org/GRAC/LigandDisplayForward?ligandId=4943
- CHMP. Blitzima: EPAR — public assessment report. 2017. Available at http://www.ema.europa.eu/docs/en_GB/document_library/EPAR_-_Public_assessment_report/human/004723/WC500233587.pdf
- CHMP. Ritemvia: EPAR — public assessment report. 2017. Available at http://www.ema.europa.eu/docs/en_GB/document_library/EPAR_-_Public_assessment_report/human/004725/WC500233388.pdf
- CHMP. Rituzena (previously Tuxella): EPAR — public assessment report. 2017. Available at http://www.ema.europa.eu/docs/en_GB/document_library/EPAR_-_Public_assessment_report/human/004724/WC500233492.pdf
- CHMP. Truxima: EPAR — public assessment report. 2017. Available at http://www.ema.europa.eu/docs/en_GB/document_library/EPAR_-_Public_assessment_report/human/004112/WC500222695.pdf
- CHMP. Amgevita: EPAR — public assessment report. 2017. Available at http://www.ema.europa.eu/docs/en_GB/document_library/EPAR_-_Public_assessment_report/human/004212/WC500225231.pdf
- CHMP. Solymbic: EPAR — public assessment report. 2017. Available at http://www.ema.europa.eu/docs/en_GB/document_library/EPAR_-_Public_assessment_report/human/004373/WC500225367.pdf
- CHMP. Imraldi: EPAR — public assessment report. 2017. Available at http://www.ema.europa.eu/docs/en_GB/document_library/EPAR_-_Public_assessment_report/human/004279/WC500233922.pdf
- CHMP. Cyltezo: EPAR — public assessment report. 2017. Available at http://www.ema.europa.eu/docs/en_GB/document_library/EPAR_-_Public_assessment_report/human/004319/WC500238609.pdf
- US Title 21CFR320.22, Criteria for waiver of evidence of in vivo bioavailability or bioequivalence. https://www.accessdata.fda.gov/scripts/cdrh/cfdocs/cfcfr/CFRSearch.cfm?fr=320.22
- https://www.ema.europa.eu/en/documents/assessment-report/tevagrastim-epar-public-assessment-report_en.pdf
- https://www.fda.gov/regulatory-information/search-fda-guidance-documents/clinical-pharmacology-data-support-demonstration-biosimilarity-reference-product
- https://www.regulations.gov/document?D=FDA-2007-P-0055-0007
- Niazi S (1976) Volume of distribution as a function of time. Journal of Pharmaceutical Sciences 65: 452—454. pmid:1263103
- Time-Varying Apparent Volume of Distribution and Drug Half-Lives Following Intravenous Bolus Injections, Wesolowski CA, Wesolowski MJ, Babyn PS, Wanasundara SN (2016) Time-Varying Apparent Volume of Distribution and Drug Half-Lives Following Intravenous Bolus Injections. PLOS ONE 11(7): e0158798. https://doi.org/10.1371/journal.pone.0158798
About The Author:
Sarfaraz K. Niazi, Ph.D., is an adjunct professor of pharmaceutical sciences at the University of Illinois and University of Houston; he has published extensively in the field of biosimilars, owns 100+ technology patents, and has provided advice to regulatory agencies on biosimilars, including the FDA. He is also actively engaged in developing biosimilars and offers consulting services to the biopharmaceutical industry.