Objectionableness: How To Know If A Microorganism Poses A Risk In Your Drug Product
By James E. Akers, Ph.D, James P. Agalloco, and Russell E. Madsen
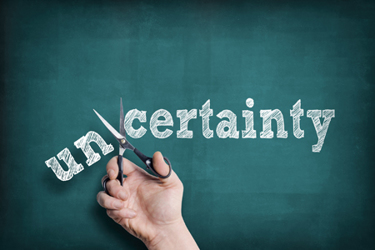
It is important to consider at the beginning of an exploration of species “objectionableness” in microbiological analysis that this idea is unique to our specific corner of the world. If you search for a definition of objectionable organisms in a microbiology reference text, you are unlikely to find one. If one puts the term “objectionable microorganism” into an internet search engine, you will find that this term is regulatory rather than scientific. In fact, it is doubtful you’ll find the word “objectionable” used as an adjective to modify the words organism or species beyond the narrow confines of the pharmaceutical/biopharmaceutical industries. Given the regulatory nature of the concept of objectionable organisms, there is virtually no scientific literature identifying key points in determining objectionableness. It is then unsurprising that efforts to develop a list of organisms that meet the definition of objectionable, while having been attempted several times, have not led to a definitive list. The development of a list was suggested during the harmonization process leading to USP <61>, <62>, and <1111>1 and their Japan Pharmacopeia and European Pharmacopeia equivalents in the 1990s. However, this effort was fraught with difficulty and ultimately abandoned. PDA Technical Report 67, Exclusion of Objectionable Organisms from Non-sterile Pharmaceuticals, Medical Devices and Cosmetics2 provided a decision tree and listed some organisms associated with recalls but did not offer a list.
Using a product recall as a reason to consider an organism objectionable is a questionable activity. The result can be a “which came first, the chicken or the egg?” argument. Was an organism considered objectionable because it resulted in a recall or was the recall predicated on a well-designed and executed risk analysis? Typically, one would need to be directly involved in the recall situation as it unfolded to know.
Pathogens And Opportunism
In the science of microbiology, organisms that are known to cause disease are technically referred to as pathogens, and thankfully they make up a tiny percentage of the microbial species with which we co-inhabit our planet. Pathogenic organisms are not necessarily deadly, and in fact, in most cases they aren’t. The common cold virus is a pathogen, but it is an annoyance rather than a killer; in epidemiological terminology if it produces a symptomatic infection also known as “morbidity”— but not death or “mortality” – it is a pathogen. SARS-CoV-2, which dominated the healthcare space in 2020, is clearly a pathogen. However, it produces symptomatic disease requiring medical attention (morbidity) in only 10% or so of those who test positive. Most of the infected people it impacts severely have preexisting health issues. True pathogens, sometimes called “frank” pathogens by microbiologists, can cause disease in otherwise healthy, uncompromised humans or animals.
So, is SARS-CoV-2 an objectionable microorganism? Certainly, anyone who experienced 2020 would answer yes, but in a drug regulatory perspective the answer would be “it depends.” SARS-CoV-2 is not environmentally resistant; in fact, it is easy to kill with most any disinfectant or cleaning agent. Soap and water at room temperature kill it quickly. It survives poorly on most metals even at room temperature. It would not survive in a hot water system for more than a few seconds. Any common pharmaceutical sterilization process would kill it instantly. It wouldn’t survive for more than an hour or two in most chemical ingredients or outside a pH range of 6 to 8. Its lipid envelope would not remain intact in the presence of even small quantities of surfactant for long. Also, a point to consider is that the common analytical methods we use to detect microbes in product would not detect any virus. Finally, it can’t be said with any certainty that SARS-CoV-2 would convey risk to any patient unless it was administered in a way that gained access to susceptible epithelial cells at numbers sufficient to cause infection, which would likely be several hundred or even a thousand plaque forming units.3
You may have heard or read the terms “opportunistic pathogen” or “opportunistic infection.” In this case, a microorganism (fungi, virus, protozoa, or bacteria) takes advantage of an opportunity not normally available to cause disease. This could include an individual with a weakened immune system, broken skin, or altered microbiome, e.g., a gut microbiota adversely affected by antibiotic therapy, to initiate an infection. It may surprise the reader to discover that even this is not a particularly long list, a few hundred species or genovars at the most. What is poorly understood is that opportunistic pathogens usually do not affect healthy humans. They could impact humans compromised by poor nutrition, poor lifestyle choices, aging, cancer, or pregnancy. Most opportunistic infections occur in persons already suffering from chronic illness and some, as is the case with cancer victims or organ transplant recipients, may be undergoing immunosuppressive therapy as a result. It is not difficult for a physician to know when a patient in their care is susceptible to opportunistic infection. It is obvious that products intended for use in at-risk patients may require more rigorous microbial attribute evaluation.
Periodically, someone asserts that opportunistic pathogens are objectionable. The answer to this is, in our opinion, a general “no.” Many opportunistic pathogens are already either part of the human microbiome or part of the human environmental microbiome, the latter referring to the environment that man inhabits, including soil, water, and domiciles.4 There is simply no way to keep such organisms out of the non-sterile products we manufacture, nor is there a way to eliminate them from our manufacturing environments. In fact, these organisms surround us constantly as we go about our daily lives.
Thus, devising a list of opportunistic pathogens and characterizing them along with true or frank pathogens as objectionable is meritless. This is because there is no effective means to screen our products or raw materials for their absence. The impracticality of analyzing statistically significant sample weights or volumes and the difficulty in taking and testing samples aseptically also work against us. It is impossible to be sure that organisms were recovered from product or are artifacts of testing when we find them in low numbers. The ubiquity of human microbiome or environmental microbiome organisms makes the possibility of false positive results high, unless the most rigorous aseptic precautions are taken. Rigorously aseptic conditions are unlikely to be found in non-sterile product manufacturing environments.
Specified Microorganisms
You may be aware of another adjective used to describe microorganisms that is also unique to the pharmaceutical industry’s regulated environment. This adjective is “specified” (and the usage of this term is most prominently associated with the compendial tests for microbiological quality of nonsterile products, for example, USP <62> Microbiological Examination of Nonsterile Products: Tests for Specified Microorganisms).
The USP contains several product monographs that require testing for the absence of certain organisms as part of the product release. These include the anaerobic spore former C. sporogenes and the aerobic organisms S. aureus, P. aeruginosa, E. coli, S. enterica serovar Typhimurium (or alternatively S. enterica serovar Abony), Aspergillus brasiliensis, and C. albicans. We have heard some industry and regulatory microbiologists refer to this list as “the magnificent seven” in calls for the expansion of this list.
Beginning in 2012 with the publication of an article titled Burkholderia cepacia: This decision is overdue, by L. Torbeck, et al,5 there has been a push to formally recognize B. cepacia complex as an “objectionable” organism. Since that time, the USP has developed chapter <60> titled Microbiological examination of non-sterile products – Test for Burkholderia cepacia complex (BCC). This chapter, which became effective in late 2019, describes an analytical method for the assessment of products for the presence of B. cepacia complex.6 However, the chapter does not designate B. cepacia objectionable. In 2017, the Microbiologics Blog conducted a survey regarding whether B. cepacia should be tested for in water systems, non-sterile pharmaceuticals, and “personal care products,” and 56% of the respondents responded yes; the remaining 44% said no.7
Objectionableness
We think B. cepacia, given the intensive and often heated discussion it has generated, is a good test case for the exploration of the condition of “objectionableness.” We believe it is a particularly good example of the considerable pitfalls associated with the broader concept of objectionableness and of how the complexity of medical microbiology makes black and white regulation, which to most people is the best kind, impossible.
Before we launch into a deeper consideration of B. cepacia, we must consider the three citations in 21 CFR 211, the current Good Manufacturing Practice regulations, that address themselves to “objectionableness” in the context of microbiological safety or purity. These citations are:
- 21 CFR 211.84(d)(6) “Each lot of a component, drug product container, or closure with potential for microbiological contamination that is objectionable in view of its intended use shall be subjected to microbiological tests before use.” (Emphasis added.)
- 21 CFR 211.113(a) “Appropriate written procedures, designed to prevent objectionable microorganisms in drug products not required to be sterile, shall be established and followed.” (Emphasis added.)
- 21 CFR 211.165(b) “There shall be appropriate laboratory testing, as necessary, of each batch of drug product required to be free of objectionable microorganisms.” (Emphasis added.)
Note that the first of these citations, 21 CFR 211.84(d)(6), appears to describe a possible condition rather a specific microorganism that might be “objectionable.” Significantly, it does link the condition of objectionableness to the way a product would be used. This appears to mean the type or condition of the patient and route of administration. The second listed citation, 21 CFR 211.113(a), requires that procedures to prevent the presence of “objectionable microorganisms” in non-sterile products “shall be established and followed.” The third citation, 21 CFR 211.165(b), requires that “appropriate laboratory testing” to show that each batch of drug product “required to be free of objectionable microorganisms” shall be done as appropriate.
Conditions Of Objectionableness
There are three obvious facts that must be determined, presumably by the manufacturer, to satisfy the requirements set forth in these regulations. First, the manufacturer must know what an objectionable organism for their product is, or at least if such a thing exists. Second, they must have a means of preventing the contamination of the product with objectionable organisms, and third, they must have a reliable analytical method able to confirm the “freedom” of a product from “objectionable microorganisms.” Note that “objectionable microorganisms” is a plural construction that means there could be more than one, or, alternatively, this can be read as suggesting that there is some quantity of microorganisms that could be considered objectionable.
Any prospective follower of this requirement must determine whether the word “freedom” means “absence of” or implies something else. The absence of a specific microorganism can’t be proved. It is impossible for any analytical method to prove a negative absolute, so we must hope that “freedom” in this regulatory context means nothing known or found. However, even if we assume freedom is meant to require zero recovery, we can’t prove absence of, because no microbiological assay has a limit of detection of zero. Also, because USP <1111> Microbial Examination of Nonsterile Products: Acceptance Criteria for Pharmaceuticals Preparations and Substances for Pharmaceutical Use states a sample volume of 1 g or 1 mL for all non-sterile product types, it would be wrong to assume that “absence of” in that small sample corresponded to “absence of” in the entire lot from which that sample was taken.
So, is this regulation truly interpretable? We’d say the only way to practically interpret it is as a well-trained microbiologist would. We certainly can’t prove “absence of”; we can only report that nothing grew, which in microbiology doesn’t mean zero. The limit of detection depends on many factors but will generally fall, depending on assay method, somewhere in the range of 50 colony forming units (CFU) to 100 to 1,000 CFU, or in scientific notation, 102 or 103 CFU.8 To further complicate things, each CFU might be one living cell or it might be 100 or 500. Our professional opinion is that if these facts were established in court, neither freedom from nor absence of could be legally enforceable. We can’t expect microbiologists or process engineers to do the impossible. Is, for example, three CFU “free” enough? We believe it should certainly be, because given the common routes of administration for most non-sterile products, the skin or mouth will be colonized and protected by millions or even billions of organisms, some of which may be of the same species a regulator might find objectionable.
The Problem With And Benefit Of Colonization
Many of the organisms that fall into the broad category of opportunistic pathogens are in fact colonizers. This is particularly true in the respiratory tract and, as we know, acute respiratory tract infections are a major cause of mortality and morbidity worldwide. Respiratory infections annually kill over a million children globally; the most common killer being the disease we call pneumonia. Pneumonia is an infection of the lower airways. The four “species” of organisms responsible for most of these infections and deaths are Streptococcus pneumonia, Haemophilus influenza, Staphylococcus aureus, and Moraxella catarrhalis.9 Fortunately, there are effective vaccines for S. pneumonia and H. influenza. So, is streptococcal pneumonia caused by a single organism, because we speak of it as one species? No! There are at least 23 strains against which conjugate vaccines have been developed and that’s only group B. There are group A S. pneumonia as well, although they are now often known as S. alginate and can cause “strep throat” as well as scarlet fever. A vaccine against group A “strep” is in development as well.
The strains listed above are colonizers and they can be transmitted to susceptible patients and in some cases cause disease. Current estimates are that 15 to 20% of school-aged children are colonized by streptococcal bacteria. These children are completely asymptomatic. About 25% of family members of these children will also have positive throat cultures for these bacteria.
Next, we turn to a charter member of the “magnificent seven” of “specified” organisms from USP <62>, which is S. aureus. This specified organism has colonized somewhere between 30% and 40% of the human race and that group is also asymptomatic for any S. aureus caused disease. To further complicate this picture, there are perhaps 1,000 strains of S. aureus and about 155,232 genetic markers.10 These strains are obviously not equally pathogenic, either in the carrier or those the carrier may infect. Most importantly though, obviously to at least one-third of the people who might take a drug with S. aureus in it, the presence of that bacterium in any number is not going to pose a risk because they are already colonized with the organism. Additionally, since such a high percentage of humans are colonized, we are in contact with these organisms on a daily basis. So, are we saving lives by rejecting a lot of tablets or lots of bulk active pharmaceutical ingredients in which we happen to recover S. aureus? No, we aren’t. Also, we must understand that because of its ubiquity in nature, the chance of false positives in recovery testing must be quite high.
You might ask, “How many bacteria do we have in our oral cavity?” There are, according to human microbiome project research, about 400 to 700 species of bacteria present in the healthy human oral cavity.11 It is impossible to know without careful analysis which of these species will have colonized a patient’s oral cavity and which are transient. There are roughly 108 to 109 microorganisms per mL of saliva and a similar number on each tooth, depending on oral hygiene.11
It is illogical to suppose that a few hundred bacteria of any kind in or on a tablet are going to make a difference to a patient’s health. Given the fact that humans are heavily colonized with enormous populations of bacteria, would rejecting a lot for finding one CFU of a potentially “objectionable” species make any difference at all to any person orally administered a drug? If we made the very conservative assumption that one mL of saliva contained only 10 million, or about 10% of the normal population of organisms in it, and a tablet had 10,000 CFU, the tablet would constitute 1/1,000 of the population of one mL of saliva in the oral cavity! Its contribution to the entire population of bacteria on the oral cavity would be even less significant.
Also, the tablet would quickly be swallowed into a digestive tract in which the stomach has a pH of 1.5 to 3.5, which is below the level most bacteria or mold can grow or even survive.14 The USP <1111> Microbial Examination of Nonsterile Products: Acceptance Criteria for Pharmaceutical Preparations and Substances for Pharmaceutical Use recommended limit for oral mucosa is 200 CFU/mL. It is impossible to believe that this recommendation is anything but extraordinarily conservative. That is 1/50 of the 10,000 CFU assumption for a tablet, above which is itself 1/5 of the maximum 2,000 CFU <1111> “limit” for a non-aqueous oral product like a tablet.
In our next article, we’ll discuss B. cepacia in more depth as a case study for determining objectionableness.
References
- USP-NF 2021 Chapters <61> Microbial Enumeration Tests, <62> Tests for Specified Organisms, and <1111> Microbial Examination of Nonsterile Products: Acceptance Criteria for Pharmaceutical Preparations and Substances for Pharmaceutical Use.
- A. Sawant, A.M. Cundell et al, 2014, PDA Technical Report Number 67 Exclusion of Objectionable Microorganisms from Nonsterile Pharmaceuticals, Medical Devices and Cosmetics.
- Transmission of SARS-CoV-2: Implications for infection and prevention precautions. WHO Scientific Brief July 9, 2020 https://www.who.int/publications/i/item/modes-of-transmission-of-virus-causing-covid-19-implications-for-ipc-precaution-recommendations.
- J. Cohen, W. Powderly, and Steven Opal. 2017 Opportunistic Infections 4th edition Vol. 1
- L. Torbeck, D. Raccasi, D. Guilfoyle, R. L Friedman and D. Hussong. 2011. Burkholderia cepacia: This Decision is Overdue. PDA J. Pharm Sci Technol. Sep-Oct 2011;65(5):535-543.
- USP-NF 2021 Chapter <60> Microbiological examination of nonsterile products- Test for Burkholderia cepacia complex (BCC).
- Kundrat, L, 2017. The Great Debate Over B. cepacia Testing: Your Opinions and FDA Recommendations. Microbiologics Blog https://blog.microbiologics.com/tag/burkholderia-cepacia-complex/
- Joanne S. Eglovitch. 2018. USP Sparks Debate Over Rapid Sterility Testing for Cell and Gene Therapies. Pink Sheet. Informa Publishing 31 Oct 2018 https://pink.pharmaintelligence.informa.com/PS124172/USP-Sparks-Debate-Over-Rapid-Sterility-Testing-For-Cell-And-Gene-Therapies
- A.A.T.M Bosch, G. Biesbroek, K. Trzcinski, E.A.M. Sanders and D. Bogeart. Viral and Bacterial Infections in the Upper Respiratory Tract. PLoS Pathogens. Published January 10. 2013. https://journals.plos.org/plospathogens/article?id=10.1371/journal.ppat.1003057
- A.van Belkum et al 2009. Reclassification of S. aureus Nasal Carriage Types, J. of Infectious Disease: Vol. 199(12); 1820-1826.
- C. M. Bassis et al. Microbiome 2, Art. No. 27, 2014. Nasal Cavity microbiota of healthy adults.https://microbiomejournal.biomedcentral.com/articles/10.1186/2049-2618-2-27
- L. Eberl and P. Vandamme, 2016. Members of the genus Burkholderia: good and bad guys. F1000Research https://f1000research.com/articles/5-1007/v1
- V.C. Scoffone et al., 2017. Burkholderia cenocepacia Infections in Cystic Fibrosis Patients Drug Resistance and Therapeutic Approaches. Front. Microbiol. Published online August 22, 2017; https://www.frontiersin.org/articles/10.3389/fmicb.2017.01592/full
- C.P. Coutinho et al. Long-term colonization of the cystic fibrosis lung by Burkholderia cepacia complex bacteria: epidemiology, clonal variation and genome-wide expression alterations. Front. Cell. Infect. Microbiol. Published Dec. 2, 2011.
About The Authors:
Jim Akers, Ph.D., is owner and principal consultant of Akers Kennedy and Assoc. He has 39 years of experience in the pharmaceutical/biopharmaceutical industry and has held various management positions in quality, development, validation, and analytics. Akers is past president of PDA and spend 15 years on its Board of Directors. He has served the USP in several capacities since 1993, including chair of the Microbiology Expert Committee, where he continues to serve as a member. Akers has also served as a technical advisor to Shibuya Corporation since 1991. He has published 36 book chapters, edited two books on Isolator technology, published over 100 articles, and lectured widely on aseptic processing, analytical microbiology, sterilization, cell processing, and advanced aseptic processing.
James Agalloco, president of Agalloco & Associates (A&A), is a pharmaceutical manufacturing expert with more than 45 years of experience. He worked in organic synthesis, pharmaceutical formulation, pharmaceutical production, project/process engineering, and validation during his career at Merck, Pfizer, and Bristol-Myers Squibb. Since the formation of A&A in 1991, Agalloco has assisted more than 200 firms with validation, sterilization, aseptic processing, and compliance. He has edited/co-edited four texts, authored/coauthored 40+ chapters, published more than 150 papers, and lectured extensively on numerous subjects. He is a past president of PDA and a current member of USP’s Microbiology Expert Committee, and serves on the Editorial Advisory Boards of Pharmaceutical Technology and Pharmaceutical Manufacturing.
Russell E. Madsen is president of The Williamsburg Group, LLC, a consulting firm located in Gaithersburg, MD. Prior to forming The Williamsburg Group, he had served PDA as acting president and was senior VP of science and technology. Before joining PDA, he was employed by Bristol-Myers Squibb as director of technical services, providing technical and general consulting services to Bristol-Myers Squibb operations worldwide. He is vice chairman of ASTM Committee E55 on Manufacture of Pharmaceutical and Biopharmaceutical Products, a member of the USP Microbiology Expert Committee, a member of Pharmaceutical Technology’s Editorial Advisory Board, and an honorary member of PDA. He holds a BS degree from St. Lawrence University and a MS degree from Rensselaer Polytechnic Institute.